Valine, isoleucine and leucine : Relationship and ratios
May. 08, 2020
- Function
- Metabolism
- Nutrition
- Swine
INTRODUCTION
Recently, with the banning of in-feed antibiotics, great attention is given on low crude protein diets supplemented with synthetic amino acids, especially on valine (Val), isoleucine (Ile), leucine (Leu). These three are categorized as branched amino acids (BCAAs). These are the most abundant of the essential amino acids (EAA) and does not only act as one of the building blocks of tissue protein, but also serve several metabolic functions (Wang et al., 2013; Zhang et al., 2017). Thirty-five to forty-five percent of the EAA in muscle are BCAAs (Ospina-Rojas et al., 2016). According to Crown et al. (2015), these BCAAs may comprise about 15-25% of total protein intake.
However, these BCAAs are metabolized using the same catabolic pathway, that is why proper balance should be taken into consideration to avoid deamination of the excess and subsequent excetion. It is very important to note that the levels of individual dietary BCAAs may affect the catabolism of all three amino acids (Hutson et al., 2005; Ospina-Rojas et al., 2016). Among the BCAAs, Leu is the most abundant from the basic raw materials used in feed formulation such as in corn and soybean meal, hence, Val and Ile supplementation becomes inevitable. Leu is present in high levels on various plant based raw materials, particularly soybean meal and corn, with 3.19% and 0.87% more than Val and Ile, respectively, causing a possible change in the ratio between the three BCAA (Vieira et al., 2016). This suggests that diets of young animals with more corn supplementation contains higher levels of Leu when compared to Val and Ile, hence the antagonistic effect of the 3 BCAA may be observed significantly at this stage.
On the other hand, BCAAs are nitrogen donors in the brain and in peripheral tissues, they are important donors for moving N in the form of alanine (Ala) and Glutamine (Gln) from muscle amino acid oxidation to the liver for urea synthesis, nitrogen (N) donors for synthesis of the excitatory neurotransmitter glutamate (Glu), and the inhibitory neurotransmitter amino butyric acid (GABA) in brain; Leu acts as an anabolic nutrient signal influencing insulin secretion by the -cells of the pancreas and protein synthesis in skeletal muscle and other selected tissues (Hutson et al., 2005). Nevertheless, BCAAs are more energy efficient than glucose and its oxidation increases under various physiological conditions to meet the energy demand including at times of starvation (Monirujjaman et al., 2014).
RELATIONSHIP
The respective catabolism enzymes of BCAAs are not restricted to the liver, instead, these are widely found in body tissues that may result to significant inter- and intra-organ exchange of BCAAs metabolites (Hutson et al., 2005). Nonetheless, BCAAs are not directly degraded in the liver and mostly metabolized in the skeletal muscle and other tissues, however, the liver can only oxidize the BCAAs after being converted to their respective -ketoacids (Zhang et al., 2017). Balancing the three BCAA is very important to avoid the catabolism of the other because of the requirements for the same enzymes: BCAA aminotransferase (BCAT) and branched-chain -keto acid dehydrogenase (BCKD) (Vieira et al., 2016). In fact, the BCAAs exert an intense antagonism between each other causing an imbalance in the plasma and brain amino acid concentration resulting to a negative effect in feed intake and weight gain in animals, hence affecting the overall feed efficiency (EFSA, 2013). This is supported by the report of Ospina-Rojas et al. (2016) that it is possible that the requirement for Val for weight gain may increase with elevated levels of Leu from the diet. An increased supply of Leu triggers the release the concentration of enzyme complex involved in the catabolism of BCAAs resulting to increased degradation of Val and Ile, since Leu stimulates BCKD that is involved in the oxidative deamination of 3 BCAA (Ospina-Rojas et al., 2016). In relation, during stressful activities, there is a normal depletion of muscle and plasma BCAAs and leads to elevated uptake of tryptophan (Trp) by brain and release of serotonin, making BCAA supplementation beneficial for body maintenance and performance (Monirujjaman et al., 2014).
Moreover, the dietary overdoses of L-Val and L-Ile are better tolerated by almost all animal species than an excess of L-Leu that may be attributed to their metabolic fate as explained by EFSA (2013). Val is a glucogenic amino acid producing its -ketoacid, -ketoisovalerate (KIV), while -keto-β-methylvalerate for Ile which is both glucogenic and ketogenic, and Leu is ketogenic with -ketoisocaproate (KIC) as the metabolite described on Fig.1 adapted from Zhang et al. (2017). These BCAA metabolites are further catabolized by a series of enzyme reactions to finally produce acetyl CoA from Leu, succinyl CoA from Val, and both acetyl CoA and succinyl CoA from Ile, which then will enter the TCA or Krebs cycle that eventually also affect the components of the blood (Ospina-Rojas et al., 2016; Zhang et al., 2017). The high concentration of Leu can stimulate the degradation of Val by the elevated intracellular levels of KIC and indirectly stimulating the BCKD complex (Ospina-Rojas et al., 2016). It is very important to note that a dietary excess of Leu could limit the availability of Val, whereas a surplus of Val could correct some of the negative effects of excessive Leu in the diet (EFSA, 2013).
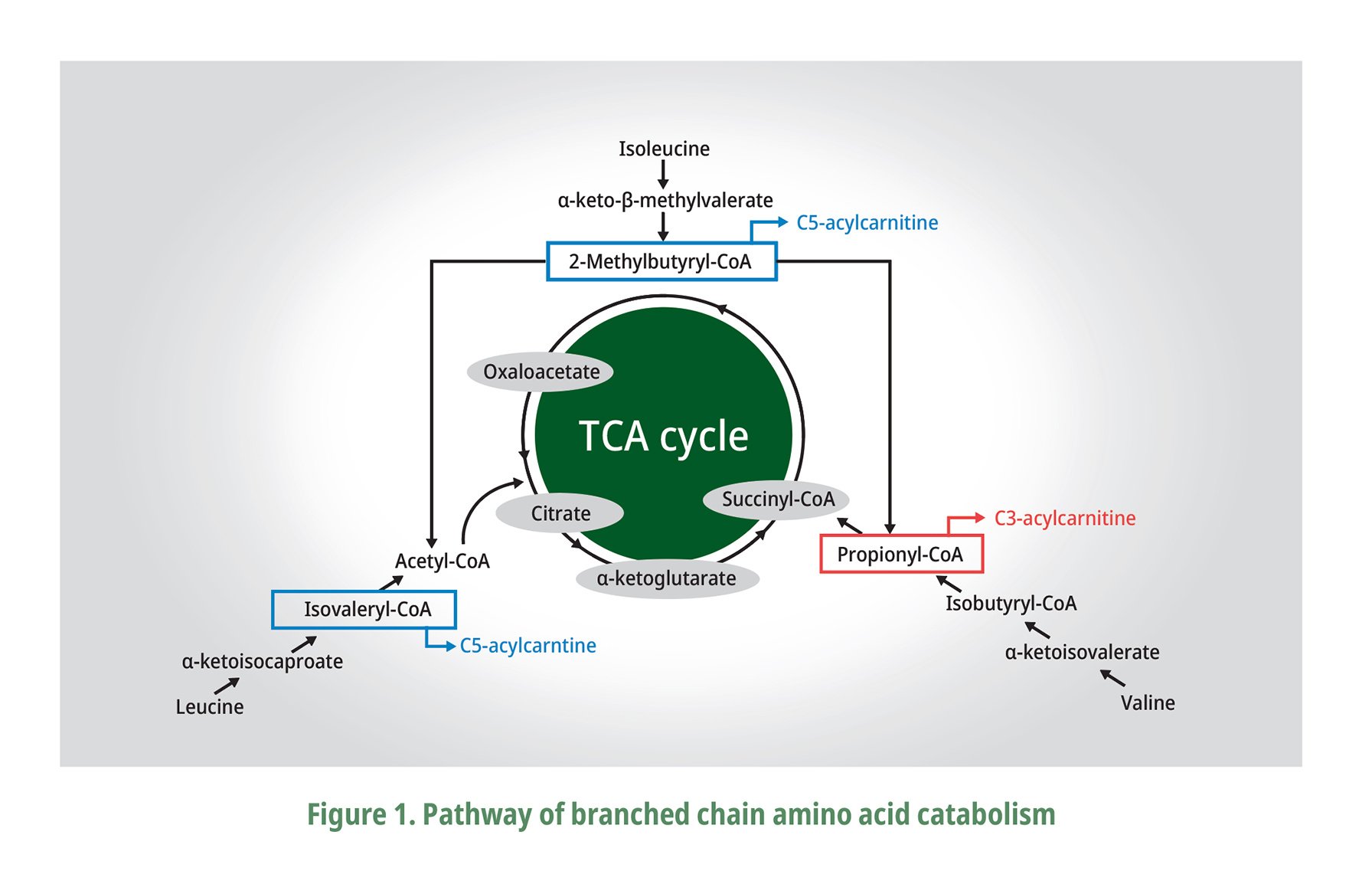
BCAA are catabolized to acetyl-CoA and/or succinyl-CoA and subsequently enter the TCA cycle. The main steps of the catabolic reactions (transamination by BCAT and decarboxylation by BCKD) are shown. With the help of BCAT, BCAA are catabolized into branched-chain -ketoacids which are subsequently decarboxylated by BCKD. Finally, all the BCAA catabolized by a series of enzyme reactions to final products and enter the TCA cycle. (Zhang et al., 2017).
RATIOS
The BCAAs can be used for maintenance of the nitrogen pools of non-essential amino acids necessary for protein synthesis to proceed. The recommended levels of branched-chain amino acids are summarized in Table 1. Ospina-Rojas et al. (2016) recently reported that weight gain in broilers fed with low crude protein diet from day 21 to 42 can be maximized with SID levels of Leu at 1.15% and Val at 0.86% representing a Val to Leu ratio of 0.75, with isoleucine level of 68% (Ile to Leu ratio of 0.59). Ospina Rojas et al. (2016) confirmed that when considering weight gain Val requirement increases with elevating levels of Leu, which signifies that the amino acid requirement needs constant updates to make up with the rapid growth rate of modern domestic animals because of continued improvement in genetic composition and selection.
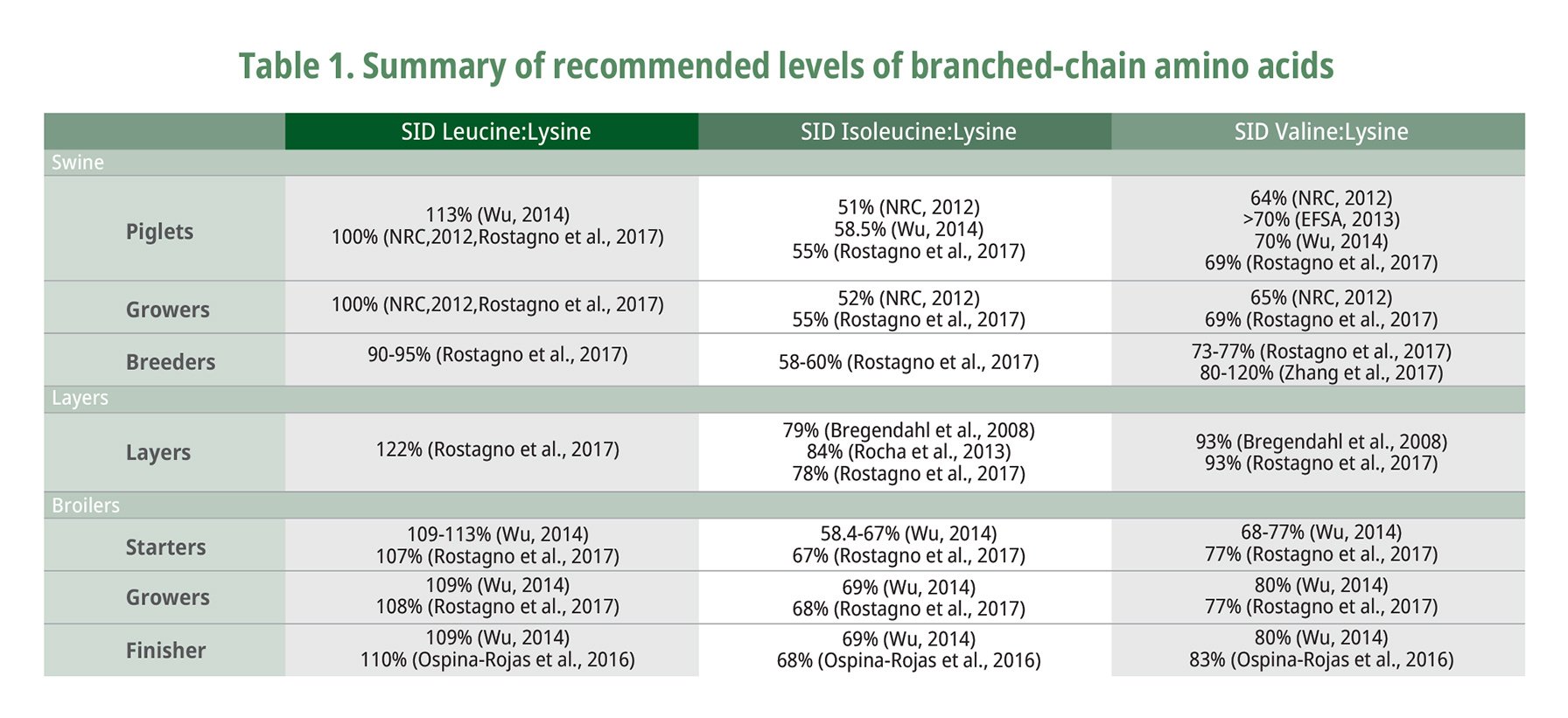
In lactating pigs, Val is very important in increasing the milk nitrogen including the synthesis of NEAA, while Leu and Ile enhance the synthesis of fractional protein (Zhang et al., 2017). For weaned piglets, Zhang et al. (2017) reported that the supplementation of BCAA in a diet with 17% crude protein at the levels of 0.1% L-Leu, 0.34% L-Val and 0.19% L-Ile improved the intestinal immune defense and increase the instestinal immunoglobulins particularly IgA and sIgA.
In layers, Rocha et al. (2013) reported that feed intake of laying hens with age 24 to 40 weeks was maximized at 86% digestible Ile relative to lysine (Lys) with Val and Leu levels of 92% and 200%, respectively. However, maximum egg mass was observed at and Ile:Lys ratio of 0.84:1 making it the optimum level of Ile for laying hens aged 24-40 weeks (Rochat et al., 2013).
REFERENCES
1. BREGENDAHL, K., S. a. ROBERTS, B. KER, and D. Hoeler. 2008. Ideal ratios of isoleucine, methionine, methionine plus cystine, threonine, tryptophan, and valine relative to lysine for white leghorn-type laying hens of twenty-eight to thirty-four weeks of age. Poult. Sci. 87-744-758.
2. Crown, S. B., N. Marze, and M. R. Antoniewicz. 2015. Catabolism of Branched Chain Amino Acids Contributes Significantly to Synthesis of Odd-Chain and Even-Chain Fatty Acids in 3T3-L1 Adipocytes. PLOS ONE 10(12):e0145850. DOI:10.1371/journal.pone.0145850.
3. DONG, X. Y., M. M. M. AZZAM, and X. T. ZOU. 2016. Effects of dietary L-isoleucine on laying performance and immunomodulation of laying hens. Poultry Science 95:2297-2305.
4. EFSA Panel of Additives and Products or Substances used in Animal Feed (FEEDAP). 2013. Scientific Opinion on the safety and efficacy of L-valine produced by the Corynebacterium glutamicum (KCCM 80058) for all animal species, based on a dossier submitted by CJ Europe GmbH. EFSA Journal 11(10):3429.
5. Hutson, S. M., A. J. Sweatt, and K. F. LaNoue. 2005. Branched-Chain Amino Acid Metabolism: Implications for Establishing Safe Intakes. J. Nutr. 135:1557S-1564S.
6. MONIRUJJAMAN, M., and A. FERDOUSE. 2014. Metabolic and physiological roles of branched-chain amino acids. Advances in Molecular Biology dx.doi.org/10.1155/2014/364976.
7. NRC. 2012. Nutrient Requirements of Swine: Eleventh Edition. National Research Council. The National Academy of Sciences. The National Academies Press. 500 Fifth Street, N. W., Washington DC.
8. OSPINA-ROJAS, I. C., A. E. MURAKAMI, C. R. A. DUARTE, G. R. NASCIMENTO, E. R. M. GARCIA, M. I. SAKAMOTO, and R. V. NUNES. 2016. Leucine and valine supplementation of low-protein diets for broiler chickens from 21 to 42 days of age. Poultry Science 0:1-9.
9. ROCHA, T. C., J. L. DONZELE, P. C. GOMES, H. H. C. MELLO, A.R. TRONI, and G. S. VIANA. 2013. Ideal digestible: digestible lysine ratio in diets for laying hens aged 24-40 weeks. Revista Brasileira de Zootecnia 42 (11):780-784.
10. Rostagno, H. S., L. F. T. ALBINO, M. I. HANNAS, J. L. DONZELE, N. K. SAKOMURA, F. G. PERAZZO, A. SARAIVA, M. L. T. DE ABREU, P. B. RODRIGUES, R. F. DE OLIVEIRA, S. L. T. BARRETO, and C. O. BRITO. 2017. Brazilian Tables for Poultry and Swine. Feedstuff Composition and Nutritional Requirements. 4th Edition. Departamento de Zootecnia, Universidade Federal de Vicosa, Brazil.
11. VIEIRA, S. L., C. STEFANELLO, and H. S. CEMIN. 2016. Lowering the dietary protein levels by the use of synthetic amino acids and the use of a mono component protease. Animal Feed Science and Technology 221:262-266.
12. WANG, C. X., and F. F. GUO. 2013. Branched chain amino acids and metabolic regulation. Biochemistry and Molecular Biology 58(11):1228-1235.
13. WU, G. 2014. Dietary requirements of synthesizable amino acids by animals: a paradigm shift in protein nutrition. Journal of Animal Science and Biotechnology 5:34.
14. Zhang, S., X. Zeng, M. Ren, X. Mao, and S. Qiao. 2017. Novel metabolic and physiological functions of branched chain amino acids: a review. Journal of Animal Science and Biotechnology 8:10.